Science > Physics > Physics and Other Sciences
Physics is a study of matter and energy in its different forms. In other words, physics is the study of nature and its laws. We expect that all the different events taking place in nature always take place according to some basic rules and revealing these rules of nature from the observed events in physics. Technology plays an important role in the benefit of society. Actually the technology is the practical application of Physics and other branches of science. Thermodynamics, a branch of physics, is evolved from the need to understand and improve the working of heat engines. The steam engine played a very important role in the Industrial Revolution in England. Physics and technology are mutually stimulated by each other; the discovery of concepts in physics is driven by technical problems, and the advancements in physics give rise to new technical problems that weren’t previously considered. Physics and technology are interrelated. It is observed that technology gives rise to new physics and at other times physics generates new technology.
1.1.4.1 Physics and Technology:
The relationship between physics and technology is deeply intertwined, with physics serving as the foundational science that underlies many technological advancements. Physics provides the principles, theories, and fundamental understanding of the natural world, and these insights are harnessed to develop new technologies that shape our daily lives. Here are key aspects of the relationship between physics and technology:
- Scientific Discoveries and Technological Innovations: Many technological breakthroughs stem from scientific discoveries in physics. For example, the understanding of electromagnetic principles laid the foundation for technologies such as radio, television, and telecommunications.
- Electronics and Semiconductor Physics: The field of electronics is built on the principles of semiconductor physics. Transistors, integrated circuits, and microprocessors are key components of electronic devices and computing systems, all rooted in the understanding of solid-state physics.
- Quantum Mechanics and Information Technology: Quantum mechanics, a branch of physics, is becoming increasingly relevant in information technology. Quantum computers, quantum cryptography, and quantum communication leverage the principles of quantum mechanics for novel computing and secure communication technologies.
- Optics and Photonics: Advances in optics, which is a branch of physics, contribute to technologies such as lasers, fiber optics, and imaging devices. These technologies are widely used in communication, medical diagnostics, and manufacturing.
- Materials Science and Engineering: Physics principles guide the development of new materials with specific properties. This is crucial for the advancement of technologies in fields such as aerospace, automotive, electronics, and renewable energy.
- Nanotechnology: Nanotechnology, which involves manipulating materials at the nanoscale, relies on principles of quantum mechanics and condensed matter physics. It has applications in medicine, electronics, materials science, and energy.
- Mechanics and Engineering: Classical mechanics, a branch of physics, provides the principles underlying the design and operation of mechanical systems, from simple machines to complex structures. It is foundational to engineering disciplines and the development of machinery.
- Thermodynamics and Energy Technologies: Thermodynamics principles are crucial for the design and operation of energy technologies, including power plants, engines, and refrigeration systems. Understanding heat transfer and energy conversion processes is essential for optimizing efficiency.
- Communication Technology: The principles of electromagnetism and information theory contribute to the development of communication technologies, including wireless communication, satellites, and the internet.
- Medical Technology: Physics plays a key role in various medical technologies, including imaging devices (X-rays, MRI, CT scans), diagnostic tools, and therapeutic technologies such as radiation therapy and laser surgery.
- Astronomy and Satellite Technology: Technologies developed for space exploration and astronomy, such as satellites, telescopes, and space probes, often involve advanced physics concepts. These technologies have practical applications in communication, navigation, and Earth observation.
- Renewable Energy Technologies: Physics principles guide the development of renewable energy technologies, including solar cells, wind turbines, and geothermal systems. Understanding the behaviour of light, heat transfer, and fluid dynamics is critical for optimizing energy conversion.
- Data Storage and Quantum Computing: Physics principles are applied in the development of data storage technologies, such as hard drives and solid-state drives. Additionally, quantum computing technologies leverage quantum mechanics to process information in ways that classical computers cannot.
- Robotics and Automation: Physics principles, especially in mechanics and control systems, are fundamental to the design and operation of robots and automated systems used in manufacturing, healthcare, and various industries.
The relationship between physics and technology is dynamic and reciprocal. Physics provides the theoretical foundation, guiding principles, and understanding of natural phenomena, while technology translates these principles into practical applications that transform and enhance various aspects of human life and industry. The synergy between physics and technology continues to drive innovation across a wide range of fields.
Physics and Technology are Supplementary to Each Other:
Physics Generating New Technology:
- Newton’s law of motion helped in the development of rockets.
- Bernoulli’s principle helped in the development of an airplane’s wings.
- The concept of thermodynamics helped in the development of heat engines.
- The heating effect of electric current helped in the development of incandescent bulbs and vacuum diodes.
- The chemical effect of electric current is used in electroplating, electrotyping, and electrorefining.
- The phenomenon of electromagnetic induction is used in electric generators, electric motors, and electric furnaces.
- The principle of conservation of energy is used in power plants.
- The theory of propagation of electromagnetic waves is applied in television, radio transmission and in wired and wireless communication.
- Digital electronics has application in computers and calculators.
- The discovery of nuclear fission has provided a tremendous source of energy. In nuclear reactors, a large amount of energy is released where mass is converted into energy. This energy is used to power generation in nuclear power plants and for destruction in a nuclear bomb.
- The phenomenon of population inversion has given rise to lasers which has very wide applications.
- The tidal energy from sea waves and solar energy is used to produce electrical energy.
Technology Generating New Physics:
- Using a discharge tube cathode rays were discovered. When cathode rays were stopped by tungsten block X-rays are produced. The discovery of x-rays helped in further development of physics. It helped in study of atomic structure, spectral analysis etc.
- Maxwell and Hertz’s work with electromagnetic waves led to the creation of wireless technology. This development of wireless technology stimulated the scientific interest in spark discharge and electrical emission. Wireless technology also led to the refinement of the theory of atoms and the development of a new theory on the states of metals.
1.1.4.2 Important scientific Principles:
Technology / Instrument/ Machine | Principle |
Steam Engine | Thermodynamics |
Aeroplane | Bernoulli’s principle |
Rocket Propulsion | Newton’s second and third law |
Hydroelectric Power | Principle of conservation of energy |
Heat Engines | Laws of Thermodynamics |
Refrigerator | Laws of Thermodynamics |
Electric Generator | Electromagnetic induction |
Radio | Electromagnetic waves |
Television | Electromagnetic waves |
Cyclotron | Simultaneous application of magnetic and electric field |
Nuclear Reactor | Nuclear Fission |
LASER | Population inversion |
Calculators | Digital logic of electronic circuit |
Computers | Digital logic of electronic circuit |
Atom Bomb | Nuclear Fission |
Hydrogen Bomb | Nuclear Fusion |
Genetic Engineering | Role of DNA in heredity |
Production of ultra-high magnetic field | Superconductivity |
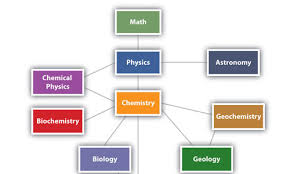
1.1.4.3 Physics and Chemistry:
Physics is useful in study of chemistry particularly in the study of atomic structure, molecular structure, X-ray diffractions, radioactivity, periodic properties of elements, nature of valency, chemical bonds in molecules, crystal structure of solids, etc.
Physics and chemistry are two closely related branches of science that share a deep connection, and they together form the foundation of physical chemistry. Here are several ways in which physics and chemistry are interrelated:
- Atomism and Molecular Structure: Both physics and chemistry contribute to our understanding of the structure of matter. The concept of atoms and molecules, fundamental to chemistry, has roots in early atomic theory and gained support through various physical experiments and models. Atomism is the philosophical and scientific idea that matter is composed of fundamental, indivisible particles called atoms. The concept of atomism has ancient roots, with early Greek philosophers such as Democritus proposing the existence of atoms around the 5th century BCE. Over time, the idea evolved and gained more acceptance, eventually forming the basis for our modern understanding of molecular structure.
- Quantum Mechanics: Quantum mechanics is a fundamental theory in both physics and chemistry. It describes the behaviour of matter and energy at the atomic and subatomic levels. It plays a central role in understanding the behaviour of matter at the atomic and subatomic levels, providing the theoretical framework for explaining the properties and interactions of atoms and molecules. In chemistry, quantum mechanics is essential for describing electronic structure, chemical bonding, molecular geometry, and spectroscopy. The quantum mechanical model of the atom, which emerged from the collaboration of physicists and chemists, laid the groundwork for understanding electronic structure and chemical bonding.
- Spectroscopy: Spectroscopy is a technique that involves the interaction of matter with electromagnetic radiation. It is used extensively in both physics and chemistry. In physics, spectroscopy helps identify the elemental composition of celestial bodies, while in chemistry, it provides information about molecular structure, chemical bonding, and electronic transitions. Spectroscopy is a powerful analytical technique that plays a crucial role in chemistry for studying the interaction of matter with electromagnetic radiation. It provides valuable information about the structure, composition, and dynamics of molecules. Various spectroscopic methods are employed in chemistry, each offering unique insights into different aspects of molecular behaviour.
- Thermodynamics: Thermodynamics is a branch of physical science that deals with the relationships between heat, work, and energy. It has applications in both physics and chemistry, providing a framework for understanding and predicting the behaviour of systems undergoing changes. The principles of thermodynamics provide a foundation for the study of physical chemistry. Thus, thermodynamics is a fundamental concept in both physics and chemistry. It provides a unified framework for understanding energy changes, heat transfer, and the spontaneity of processes in diverse systems, ranging from chemical reactions to heat engines.
- Statistical Mechanics: Statistical mechanics is a bridge between physics and chemistry that explains macroscopic thermodynamic behaviour in terms of the statistical properties of microscopic particles. It is used to derive thermodynamic laws from the behaviour of individual particles, contributing to our understanding of the behaviour of gases, liquids, and solids.
- Chemical Kinetics: Chemical kinetics is the branch of chemistry that deals with the study of reaction rates, mechanisms, and the factors affecting the speed of chemical reactions. It explores how quickly or slowly chemical reactions occur and the factors that influence the rates of these reactions. Key concepts in chemical kinetics include reaction rates, rate laws, reaction mechanisms, and reaction orders. The study of reaction rates, is an area where physics concepts, such as collision theory, are applied to understand the mechanisms and dynamics of chemical reactions. The rates of chemical reactions can be explained using principles from classical mechanics.
- Electrochemistry: Electrochemistry explores the relationship between chemical processes and electrical energy. It involves the study of redox reactions and the behaviour of ions in solution. Physics principles, particularly those related to electrical circuits and conductance, are applied to understand electrochemical phenomena.
- Materials Science: Physics and chemistry collaborate in the field of materials science, where the properties and behaviours of materials are studied. Understanding the structure-property relationships of materials involves both chemical considerations (composition, bonding) and physical considerations (electronic structure, crystallography).
- Physical Organic Chemistry: Physical organic chemistry integrates principles from both physics and chemistry to study the relationship between molecular structure and reactivity. It investigates how the electronic and steric factors influence the mechanisms and rates of organic reactions.
The relationship between physics and chemistry is intimate and pervasive. They share common principles and methodologies, and advancements in one field often contribute significantly to the other. Physical chemistry, as a discipline, specifically focuses on the intersection of physics and chemistry, providing a comprehensive understanding of the principles that govern the behaviour of matter.
1.1.4.4 Physics and Biology:
The discovery of optical microscope or electron microscope helped biology in studying the microorganisms and the structure of cells. X-rays are used to study defects, fractures in human body. Ultrasonography is used to study inner organs. Radiography is used for treatment of cancer etc. Physics and biology are two distinct scientific disciplines, but they are interconnected and share fundamental principles. The relationship between physics and biology is evident in several ways:
- Biophysics: Biophysics is a scientific discipline that sits at the intersection of physics and biology. It involves the application of physical principles and methods to study biological systems, aiming to understand the mechanisms and processes that govern life at various levels of organization, from the molecular to the organismal. Biophysics integrates the principles and methodologies of physics with the complexity of biological systems. It provides a quantitative and rigorous approach to understanding life processes, offering insights that are essential for advancing both physics and biology. The interdisciplinary nature of biophysics contributes to advancements in medical research, biotechnology, and our overall understanding of the fundamental principles underlying living organisms.
- Molecular Biology: Molecular biology is a branch of biology that focuses on the study of biological processes at the molecular level. It involves the understanding of the structure and function of biomolecules, the mechanisms of molecular interactions, and the regulation of various cellular processes. Understanding these mechanisms often involves concepts from physics and chemistry, such as the behaviour of biomolecules, molecular interactions, and the structure and function of macromolecules like DNA, RNA, and proteins. Thus, molecular biology provides a detailed understanding of the fundamental processes that govern life at the molecular level. Its insights have profound implications for medicine, genetics, biotechnology, and our overall understanding of the molecular basis of living organisms.
- Thermodynamics: Thermodynamics, a branch of physics, plays a crucial role in understanding energy transfer and transformation in biological systems. Thermodynamics plays a crucial role in understanding and describing various processes within biological systems. The principles of thermodynamics provide a framework for analyzing energy transfer and transformation, as well as the spontaneity and efficiency of biochemical reactions. Thermodynamics is essential for unravelling the energetics of cellular processes, predicting the feasibility of biochemical reactions, and gaining insights into the efficient utilization of energy in living organisms. It provides a foundation for studying metabolism, cellular respiration, and other fundamental processes that sustain life.
- Quantum Biology: Quantum biology is an interdisciplinary field that explores the application of quantum mechanics principles to biological systems. While classical physics effectively describes many macroscopic phenomena, quantum biology investigates whether quantum effects play a role in the behaviour of biological molecules and processes at the microscopic level. It is important to note that quantum biology is a relatively young and evolving field, and some of its claims and hypotheses are still a subject of debate and ongoing research. While there is evidence suggesting quantum effects in certain biological processes, the extent to which quantum mechanics is relevant to the overall functioning of living organism remains an open question. Quantum biology represents a fascinating intersection between quantum physics and the complexity of biological systems, and it continues to inspire new avenues of research and exploration.
- Neurophysics: Neurophysics is an interdisciplinary field that applies the principles and techniques of physics to study the structure and function of the nervous system. It seeks to understand the physical mechanisms that underlie neural processes, from the level of individual neurons to complex neural networks. Neurophysics seeks to bridge the gap between physics and neuroscience, using the tools and concepts of physics to gain insights into the fundamental principles governing the structure and function of the nervous system. This interdisciplinary approach is essential for advancing our understanding of brain function and for developing new technologies for both basic research and clinical applications.
- Biomechanics: Biomechanics is the study of the mechanical aspects of living organisms, including their structure, function, and motion, using principles from physics and engineering. It applies the laws and methods of mechanics to understand how biological systems move, respond to forces, and maintain their structural integrity. Biomechanics plays a vital role in advancing our understanding of the mechanical aspects of living organisms. It has applications in various fields, including medicine, sports science, rehabilitation, ergonomics, and orthopaedics, contributing to the development of interventions and technologies that improve human health and performance
- Electrophysiology: Electrophysiology is the branch of physiology that studies the electrical properties of biological cells and tissues. It involves the measurement and analysis of electrical currents and voltages generated by physiological processes. Electrophysiological techniques are widely used to understand the function of cells, organs, and entire organisms. Electrophysiology is a versatile and essential field that provides valuable insights into the electrical aspects of biological systems. It has broad applications in neuroscience, cardiology, muscle physiology, and clinical diagnostics, contributing to our understanding of normal physiology and the mechanisms underlying various diseases.
- Statistical Physics and Evolution: The connection between statistical physics and evolution is an interdisciplinary area that explores how principles from statistical physics can be applied to understand evolutionary processes. While classical Darwinian evolution relies on natural selection acting on individual organisms based on their traits, statistical physics provides a framework for describing the collective behaviour of large populations. The application of statistical physics to evolution provides a mathematical and computational framework for understanding the dynamics of genetic variation and adaptation within populations. It helps explore the role of chance, randomness, and collective behaviours in shaping evolutionary trajectories and patterns of biodiversity. The interdisciplinary nature of this field contributes to a deeper understanding of the complexity of evolutionary processes.
The relationship between physics and biology is multifaceted, with principles from physics providing a foundation for understanding the physical and molecular processes that govern living organisms. The interdisciplinary nature of these fields allows scientists to apply tools and concepts from physics to gain deeper insights into the complexities of biological systems.
1.1.4.5 Physics and Medicine:
The relationship between physics and medicine is profound, and physics plays a crucial role in various aspects of medical science and healthcare. The application of physics principles and technologies in medicine has led to advancements in diagnostics, imaging, treatment, and research.
- X-ray Imaging: Physics principles, particularly those related to electromagnetic radiation, are fundamental to X-ray imaging. X-rays are used for diagnostic purposes, such as detecting fractures, tumors, and assessing the condition of internal organs.
- Magnetic Resonance Imaging (MRI): MRI relies on principles of nuclear magnetic resonance, a phenomenon in quantum mechanics. Magnetic fields and radiofrequency pulses are used to create detailed images of soft tissues, providing valuable information for diagnosis.
- Computed Tomography (CT): CT scans use X-rays and principles of tomography to create cross-sectional images of the body. Physics guides the design of CT scanners and the interpretation of images.
- Radiation Therapy: Physics is essential in the field of radiation oncology for cancer treatment. Radiation therapy uses ionizing radiation to target and destroy cancer cells. Accurate dose delivery and treatment planning involve sophisticated physics principles and technologies.
- Ultrasound Imaging: Ultrasound imaging utilizes principles of acoustics. High-frequency sound waves are transmitted into the body, and the echoes are used to create images of internal structures. Physics guides the interpretation of ultrasound images and the design of ultrasound equipment.
- Nuclear Medicine: Nuclear medicine involves the use of radioactive materials for diagnostic and therapeutic purposes. Physics principles, such as radioactive decay and detection methods, are central to procedures like positron emission tomography (PET) scans and radioiodine therapy.
- Biophysics: Biophysics applies physics concepts to study biological systems. Understanding the physical properties of biological molecules, cellular processes, and biomechanics is crucial for advancing knowledge in areas such as physiology, pharmacology, and neuroscience.
- Medical Instrumentation: Physics principles guide the development of medical instruments and devices. Technologies like electrocardiography (ECG), electroencephalography (EEG), and medical lasers are examples of applications where physics is integral to device functionality.
- Dosimetry and Radiation Safety: Physics is essential for measuring and monitoring radiation doses in medical procedures. Dosimetry ensures that patients receive the prescribed dose in radiation therapy while minimizing exposure to healthy tissues. Physics principles also guide radiation safety protocols for healthcare professionals.
- Magnetic Resonance Spectroscopy (MRS): MRS, an extension of MRI, measures the concentration of certain biochemical compounds in tissues. It provides insights into cellular metabolism and is used in research and clinical settings.
- Medical Physics Research: Physicists engage in medical research to develop new technologies and improve existing ones. Research areas include the development of advanced imaging techniques, novel treatment modalities, and innovative diagnostic tools.
- Biomedical Engineering: Biomedical engineers often apply physics principles to design medical devices and technologies. This includes the development of prosthetics, medical imaging systems, and diagnostic equipment.
- Health Monitoring and Wearable Devices: Physics-based sensors and technologies are employed in wearable devices for health monitoring. Examples include accelerometers for activity tracking and biosensors for measuring physiological parameters.
- Drug Delivery and Nanomedicine: Physics principles are applied in drug delivery systems, including the design of nanoparticles for targeted drug delivery. Understanding the behavior of particles at the nanoscale is crucial for developing effective therapeutic strategies.
The integration of physics and medicine continues to drive advancements in healthcare, leading to improved diagnostics, personalized treatments, and enhanced patient care. The collaboration between physicists, engineers, and medical professionals is essential for pushing the boundaries of medical science and technology.
1.1.4.6 Physics and Astronomy:
Galileo developed first optical telescope. It is used for studying distant planets. Giants telescope by physics are used to study stars, galaxies etc. Radio telescope helped in discovery of pulsars and quasars.
Physics and astronomy are closely related disciplines, with physics serving as the foundational science that underpins much of our understanding of the universe. The relationship between physics and astronomy is intricate, as both fields share common principles and methodologies. Here are key aspects of their interconnection:
- Fundamental Laws of Physics: The laws of physics, including Newton’s laws of motion, gravitation, and the laws of thermodynamics, provide the basic principles governing the behaviour of matter and energy. These laws apply universally, forming the foundation for understanding celestial bodies and their interactions.
- Celestial Mechanics: Celestial mechanics, a branch of physics, applies the principles of classical mechanics to the motion of celestial bodies. Newton’s law of gravitation is fundamental in describing how planets, stars, and other celestial objects move within gravitational fields.
- Gravitational Astronomy: Einstein’s general theory of relativity, a cornerstone of modern physics, extended and refined our understanding of gravity. Gravitational astronomy explores phenomena such as gravitational waves, which are ripples in spacetime caused by the acceleration of massive objects.
- Astrophysics: Astrophysics integrates principles from physics to study the properties and behaviour of celestial objects. This includes the study of stellar structure and evolution, the behaviour of galaxies, and the properties of the interstellar medium. The laws of thermodynamics are particularly relevant in understanding processes within stars and galaxies.
- Spectral Analysis: Physics-based techniques, such as spectroscopy, are widely employed in astronomy. Spectral analysis allows astronomers to determine the composition, temperature, density, and motion of celestial objects by studying the light they emit or absorb.
- Nuclear Physics and Stellar Fusion: Nuclear physics principles are crucial in understanding stellar processes, such as nuclear fusion reactions that power stars. The study of stellar nucleosynthesis, which involves the synthesis of elements within stars, relies on nuclear physics concepts.
- Cosmology: Cosmology, the study of the large-scale structure and evolution of the universe, relies heavily on physics. The application of general relativity, thermodynamics, and quantum mechanics contributes to our understanding of the cosmos on the grandest scales.
- Particle Astrophysics: Particle physics principles are applied in astrophysics to study high-energy particles originating from celestial sources. Cosmic rays, high-energy photons, and neutrinos are investigated to understand the extreme conditions in the universe.
- Dark Matter and Dark Energy: Physics plays a key role in addressing the mysteries of dark matter and dark energy, which together constitute a significant portion of the universe. Understanding their nature requires the application of particle physics and cosmological principles.
- Observational Techniques: Physics-based instruments and techniques, such as telescopes, detectors, and imaging devices, are crucial in observational astronomy. Advances in physics contribute to the development of cutting-edge instruments that enhance our ability to explore the universe.
- Astroinformatics: Computational methods and data analysis techniques from physics are increasingly employed in the emerging field of astroinformatics. This involves handling large datasets, simulations, and complex modelling to extract meaningful information from astronomical observations.
- Space Exploration: Physics plays a central role in the design and operation of spacecraft and probes for space exploration. The principles of mechanics, electromagnetism, and thermodynamics are applied in creating and navigating space missions.
The relationship between physics and astronomy is symbiotic, with physics providing the theoretical and experimental framework for understanding the fundamental laws that govern the universe. The interdisciplinary nature of the two fields enhances our ability to explore and comprehend the complexities of the cosmos.
1.1.4.7 Physics and Mathematics:
The relationship between physics and mathematics is deep and fundamental. Mathematics serves as the language of physics, providing the tools and framework to formulate theories, express relationships, and make predictions about the physical world. Here are several aspects of the intricate connection between physics and mathematics:
- Descriptive and Predictive Power: Mathematics enables physicists to describe physical phenomena precisely and make predictions about the behavior of systems. Equations and mathematical models are used to express the fundamental laws of nature, guiding our understanding of the physical universe.
- Formulation of Physical Laws: Physical laws, such as Newton’s laws of motion, Maxwell’s equations for electromagnetism, and Einstein’s equations of general relativity, are formulated mathematically. Mathematics allows the expression of complex relationships in concise and elegant forms, facilitating the development of theoretical frameworks.
- Quantitative Analysis: Mathematics provides the tools for quantitative analysis and measurement. The use of mathematical equations allows scientists to quantify physical quantities, predict outcomes, and compare observations with theoretical expectations.
- Mathematical Modelling: Physicists use mathematical models to represent real-world phenomena. These models, often expressed as differential equations or other mathematical structures, capture the essential features of a system and enable predictions and simulations.
- Symmetry and Conservation Laws: The concept of symmetry in mathematics plays a crucial role in physics. Symmetry principles, such as Noether’s theorem, connect symmetries with conservation laws, revealing profound connections between mathematical structures and physical quantities like energy, momentum, and angular momentum.
- Calculus and Dynamics: Calculus is a fundamental branch of mathematics used extensively in physics. It provides tools for understanding rates of change, motion, and the accumulation of quantities. Differential equations, integral calculus, and concepts like limits are essential in describing dynamic systems.
- Vector Spaces and Linear Algebra: Vector spaces and linear algebra are foundational in physics. They are used to represent physical quantities with magnitude and direction (vectors) and describe linear transformations, quantum states, and other mathematical structures.
- Complex Numbers in Quantum Mechanics: Complex numbers play a central role in quantum mechanics. Wave functions, probability amplitudes, and quantum operators are often expressed using complex numbers, providing a powerful mathematical framework for understanding the quantum realm.
- Statistical Methods and Probability Theory: Probability theory and statistical methods are employed in physics to describe uncertainty, randomness, and the behavior of large ensembles of particles. These mathematical tools are essential in statistical mechanics, quantum mechanics, and cosmology.
- Group Theory in Particle Physics: Group theory, a branch of abstract algebra, is extensively used in particle physics. Symmetry groups and representations play a key role in classifying particles and understanding the fundamental forces in the Standard Model of particle physics.
- Topology and Field Theory: Topology is applied in the study of phase transitions and defects in materials, while field theory, a branch of mathematics, is crucial in theoretical physics. The mathematical framework of field theory is used in quantum field theory, which describes the fundamental forces and particles in the universe.
- Mathematical Rigor and Clarity: Mathematics provides a level of rigor and clarity in expressing physical theories. Mathematical formulations allow for precision, logical deduction, and the development of a unified and consistent theoretical framework.
The relationship between physics and mathematics is symbiotic. Physics relies on the language of mathematics to formulate theories, model physical systems, and make predictions. Conversely, the challenges posed by physical phenomena often drive the development of new mathematical concepts and techniques, leading to a continual exchange and enrichment of both disciplines.
1.1.4.8 Physics and Society:
Society’s reliance on technology represents the importance of physics in daily life. Many aspects of modern society would not have been possible without the important scientific discoveries made in the past. These discoveries became the foundation on which current technologies were developed.
Physics, as a fundamental branch of science, has profound and far-reaching impacts on society. The relationship between physics and society is multifaceted, encompassing technological advancements, medical breakthroughs, environmental understanding, and contributions to our daily lives. Here are several ways in which physics influences society:
- Technological Advancements: Physics is at the core of technological innovations. Advances in semiconductor physics have led to the development of computers, smartphones, and other electronic devices. Fields like quantum physics and materials science drive progress in emerging technologies, including quantum computing and advanced materials.
- Energy Production and Consumption: Physics plays a crucial role in energy-related issues. Understanding the principles of thermodynamics, electromagnetism, and nuclear physics is essential for the generation, distribution, and efficient use of energy. Renewable energy technologies, such as solar panels and wind turbines, rely on principles of physics.
- Medical Imaging and Diagnosis: Medical physics contributes to advancements in diagnostic imaging techniques, such as X-rays, magnetic resonance imaging (MRI), and computed tomography (CT). These technologies allow for non-invasive visualization of internal structures, aiding in medical diagnosis and treatment.
- Communications and Information Technology: The field of optics and electromagnetism underlies the development of communication technologies, including fiber optics, lasers, and telecommunications. Information theory, a branch of physics, forms the basis for data compression, encryption, and transmission.
- Transportation: The physics of motion and fluid dynamics play a role in transportation technologies. Aerodynamics is crucial for designing efficient aircraft, while principles of mechanics and thermodynamics contribute to the design of automobiles and engines.
- Environmental Science: Physics is integral to understanding environmental phenomena. Climate science relies on principles of thermodynamics, fluid dynamics, and radiative transfer. Physics also contributes to environmental monitoring technologies and the study of pollution and climate change.
- Materials Science and Engineering: Advances in materials science, guided by principles of physics, have led to the development of new materials with unique properties. This impacts industries such as electronics, construction, aerospace, and healthcare.
- Scientific Research and Innovation: Physics drives scientific research and innovation across disciplines. Technologies such as particle accelerators and synchrotrons contribute to fundamental research in physics, chemistry, biology, and materials science.
- Educational Impact: Physics education fosters critical thinking, problem-solving skills, and a deeper understanding of the natural world. It contributes to a scientifically literate society, shaping the perspectives and decisions of individuals in various fields.
- Space Exploration: Physics is fundamental to space exploration and our understanding of the universe. Technologies developed for space missions have practical applications on Earth, and space research contributes to advancements in astrophysics and cosmology.
- Social and Ethical Considerations: Physics, especially in fields like nuclear physics and artificial intelligence, raises social and ethical considerations. Society grapples with the responsible use of technology, environmental impacts, and ethical considerations associated with scientific research.
- Global Challenges: Physics contributes to addressing global challenges, including sustainable development, clean energy solutions, and mitigating the impact of natural disasters. Scientific collaboration and the application of physics principles are crucial for finding solutions to pressing global issues.
The relationship between physics and society is dynamic and reciprocal. Physics provides the tools and knowledge that shape technological progress, influence daily life, and contribute to societal well-being. Conversely, societal needs and challenges drive the pursuit of new avenues in physics research and application. The intersection of physics and society is a key driver of progress in science, technology, and the improvement of human conditions.
From above explanation we can conclude that world has come closer and standard of living is increased. But at the same time it created some problems in the society.
- With knowledge physics, an atom bomb is developed. The atomic bomb explosion at Hiroshima and Nagasaki killed thousands of people many became physically disabled.
- Constant use of energy resulted in the problem of global warming and the greenhouse effect.
- Continuous use of technology from day to night made our life lazy.
Conclusion:
Physics, often referred to as the “fundamental science,” plays a central role in influencing and interacting with various other scientific disciplines. The relationship between physics and other sciences is intricate, with physics providing foundational principles and concepts that are applied and extended in interdisciplinary research. The relationship between physics and other sciences is interdisciplinary and mutually enriching. Physics provides a fundamental framework for understanding the natural world, and its principles are applied across diverse scientific domains, fostering collaboration and advancing knowledge across disciplinary boundaries.
Related Topics:
3 replies on “1.1.4 Physics and Other Sciences”
Absloutely amazing information
Wow great
value able informatin